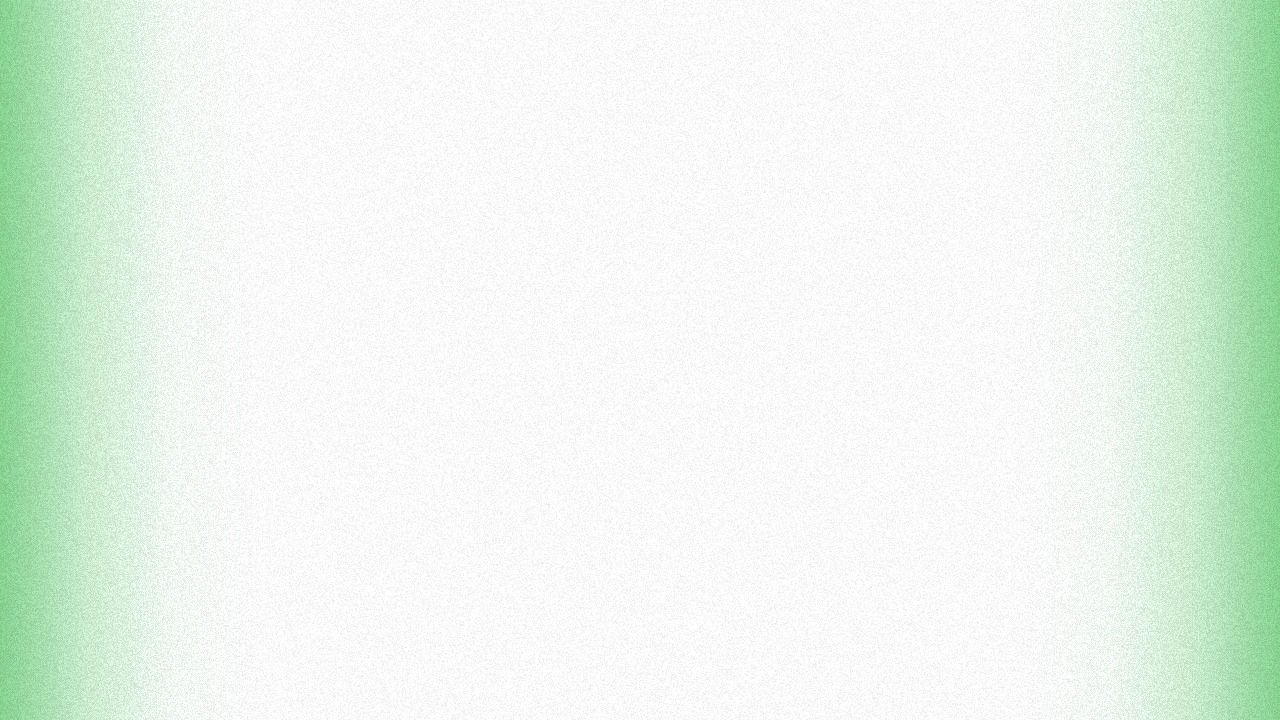
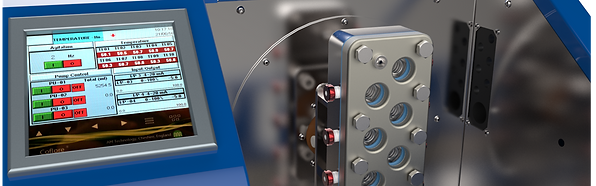
This site uses cookies. Click here to find out more.
Flow Chemistry
Overview of Flow Chemistry
What is Continuous Flow Chemistry?
Continuous flow chemistry, also known as flow chemistry, conducts chemical reactions in a continuously flowing stream. A continuous flow chemistry system ensures that production is achieved by starting materials entering the reactor at the same rate the product exits.
How is Flow Chemistry, Continuous?
This seamless process is continuous flow chemistry. As a continuous chemical manufacturing method, the flow of reagents and raw materials is continuously pumped through the reaction site, at a lower working volume than batch, to ensure safety and 'on-demand' production. This is also referred to as operating at "steady state" to produce material continuously and indefinitely without interruption.
It is this continuous production at "steady-state" that is the main differentiator between continuous flow chemistry and batch production, the other widely used method of chemical production. Flow reactors employ flow chemistry principles to provide continuous chemical manufacturing capabilities to a variety of industries including pharmaceutical and chemical,
The continuous flow chemistry reaction pathways used in flow reactors are typically tube-like in design and are manufactured from non-reactive materials such as Hastelloy or silicon carbide. The mixing methods in flow reactors can include diffusion alone, passive (or static) mixing and, more recently, active mixing (also described as dynamic mixing).
Why is Flow Chemistry Important?
Continuous flow technology brings versatility, productivity and efficiency to chemical manufacturing. By enabling precise control over important reaction parameters, such as heat transfer (heating and cooling), mixing (and mass-transfer) and reaction time (residence time), flow chemistry provides significant benefits in terms of safety.
Such a level of control also brings with it better potential outcomes of reaction processes, including percentage yields, purities and selectivities, whilst providing low capital costs, small reactor footprints, efficient energy use, reduced solvent use, low emissions and improved safety management.
Additionally, the concept of operating at a period of steady-state, as is the case with continuous flow reactors, typically reduces the number of operator interventions required in a chemical production plant compared to batch-wise production.
Advantages of Flow Chemistry
There are well-defined key advantages to using flow technologies as compared to standard batch chemistry methods:
-
Improved heat transfer
-
Improved mass transfer/mixing
-
Reproducibility
-
Easier route to scale-up
-
Multistep (telescoping)
-
In-line downstream processing
-
Automation
-
Improved Safety (managing hazardous reagents and intermediates)
-
Smaller reactor footprint
-
Lower operational expenditure or running costs
-
Lower fugitive emissions
-
Lower solvent use and waste
Many of the benefits of continuous flow technology also address the principles of green chemistry. Flow chemistry delivers more sustainable and safer chemical processes, further details can be found in our recent article in the Chemical Industry Journal.
Batch Chemistry vs Flow Chemistry
-
Versatile; a batch reactor can be easily adapted to handle many different processes and campaigns.
-
Productive; large amounts of material can be produced with each batch.
-
Established; batch technology is well known within the chemical industry and a vast number of the process have been designed and used in batch.
However, batch processing proceeds much more slowly and as such the overall cost of processing increases by comparison with flow chemistry. Start-up and shut-down processes of batch equipment can increase energy consumption and waste material, and the quality discrepancy between batches can be significant. This can lead to lost production and compromised quality if the batch process isn’t monitored closely.
Flow chemistry has one main disadvantage, which is that overall, batch processing remains the standard within the fine chemical and pharmaceutical industries, simply because versatility, productivity and established know-how are extremely desirable attributes when considering a route to chemical manufacture. Despite the many additional advantages and efficiencies of flow chemistry over batch.
Flow technologies that embrace and build upon versatility and productivity will open up the possibility for their widespread use within the chemical industries. This is the motivation and rationale for the design of our range of Coflore flow reactors from AM Technology.
The range can be used independently for proof of concepts or our continuous flow technology can be used to build production scale skids, transitioning a batch process into an end-to-end continuous process. Check out how flow chemistry is used to build a process skid below!
What are the Different Types of Flow Chemistry Reactors?
There are a number of types of reactors in flow chemistry, including plug flow reactors and continuous stirred tank reactors. Delve deeper into how continuous flow technology works by looking at the various types of flow chemistry reactors:

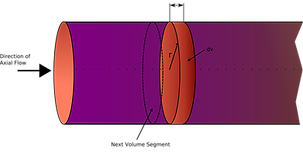

